The injection of cryogenic ice cubes is the method that will be used in ITER to feed the plasma with fuel. The CEA-IRFM, in collaboration with the National Institute for Fusion Science (NIFS) in Japan, has made advances in understanding and simulating the ablation of ice cubes in fusion plasmas.
In fusion power plants, fuel must be injected continuously to maintain the fusion reaction. Injecting ice helps to control the density of the plasma by compensating for the loss of particles, whether they have fused, been trapped in the walls of the machine or evacuated to the pumps before they have reacted.
The simplest method of supplying fuel to the plasma consists of injecting gas through orifices in the wall of the tokamak, connected by tubes to piezoelectric microvalves placed outside the vacuum chamber. However, due to the high density and temperature of the plasma, the neutral atoms injected in this way are rapidly ionised and cannot penetrate deep into the core of the plasma where the fusion reactions take place. To overcome this limitation, fuel is injected in solid form, propelled at high speed: this is the injection of deuterium and tritium ice cubes, at speeds of up to a few km/s. At the CEA, the IRIG is a major player in the development of these ice injectors.
The HPI2 code, developed by CEA-IRFM and the Austrian OAW Institute, simulates the penetration of the ice cube into the plasma and the drift of the ice particles, which are gradually sublimated and then ionised by collisions with the electrons and hot ions in the plasma. Various models exist to describe this complex physics. Work carried out at the CEA-IRFM has validated certain hypotheses of the HPI2 model, by comparing its results with detailed visible spectroscopy measurements, in particular those carried out on Tore Supra (CEA tokamak now transformed into WEST) and more recently on the LHD (Large Helical Device) stellarator, located at the National Institute for Fusion Science (NIFS) in Gifu, Japan.
One of the most remarkable phenomena is the observation of striations when the ice cube is ablated during its penetration. The photograph taken on Tore Supra during the penetration of the ice cube into the plasma (Figure 1a) shows a discontinuous deposit of material correlated with observations of oscillations in the intensity of the first spectral line emitted by the deuterium ions (Dalpha signal) measured by visible spectroscopy (Figure 1b).
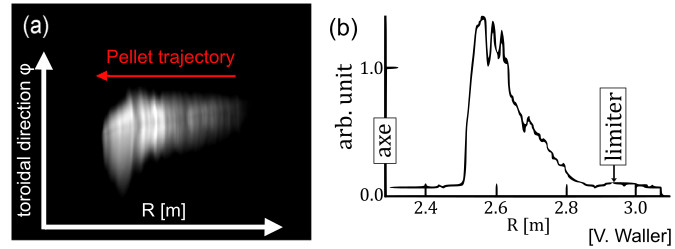
This phenomenon is interpreted as follows: when ice at -250°C comes into contact with plasma at several tens of millions of °C, its surface is rapidly heated and vaporised by collisions with ions and electrons from the plasma. A cloud of neutral gas then surrounds the ice cube, forming a shield that reduces the flow of particles from the plasma. This cloud will gradually increase in temperature and become partially ionised. It will also expand spatially, as shown in Figure 1a by the gradual increase in the toroidal extent of the light emitted by the cloud along the trajectory of the ice cube. The ionised part, which is very dense, will undergo the drift phenomena typical of magnetised plasmas, leading to its detachment from the ‘ice cube + neutral cloud’ system. This plasmoid will homogenise in the surrounding plasma: it is the plasma’s fuel source. The ice cube is then once again surrounded only by a neutral cloud and the process begins again: ionisation, drift, homogenisation, until the ice cube has been completely vaporised.
The HPI2 code models this cyclical phenomenon, enabling a detailed comparison with experiment. The code’s predictions have been compared with well-documented measurements of ice cube injection on the LHD stellarator. While the particle content of the cloud and the frequency of striations are a function of the position of the ice cube, the radius and length of the cloud change continuously with time. The measurement points obtained from the experimental images depend on the camera dynamics and only the main trends can be compared. As shown in Figure 2, the average striation frequency predicted by the code (of the order of 100kHz) is in good agreement with the experimental measurements, both in terms of amplitude and variation along the trajectory of the ice cube. The other properties of the cloud are also correctly validated along its trajectory: its size, its ablation rate, etc.
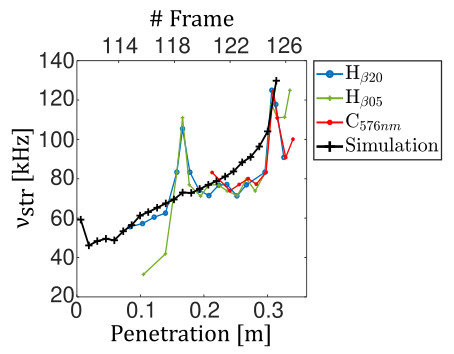
The good agreement between simulations and experimental observations, at an unprecedented level of detail, is another important milestone in the validation of the HPI2 code. It confirms the relevance of the approximations used to describe the physical ablation processes. The ability of the code to be applied to different magnetic fusion systems (tokamak, stellarators, etc), as well as its flexible interfacing with other numerical tools, now make it a module of choice for the operational preparation of new fusion projects: ITER of course, but also the European DEMO project and several projects from the private sector. However, the certainty that the code will adequately describe the source of matter by ice cube injection in these new machines is not completely guaranteed. The conditions will not be the same as in the current machines, and this ability to extrapolate outside known conditions can only be strengthened by continuing the validation process in the new installations.
Publication
Structure of pellet cloud emission and relation with the local ablation rate, B. Pégourié et al. 2024 Nucl. Fusion 64 056026, DOI 10.1088/1741-4326/ad326c