Several key physical issues for the successful operation of ITER (International Thermonuclear Experimental Reactor) are related to the tokamak plasma edge turbulence. First of all, the excess power produced by fusion in the confined plasma must be disposed of through a relatively small available surface. The associated heat fluxes are close to the threshold above which thermo-mechanical stresses deform, and possibly melt, the recipient material. Electrostatic turbulence, one of the main transport mechanisms in tokamak plasma edge, strongly affects the surface available for the heat flux draining. Indeed turbulence regulates the outflow of particle and heat fluxes from the confined region towards the one closest to the solid walls. Experimental investigations on this problem are the core subject of the WEST (tungsten (W) Environment in Steady-state Tokamak) at IRFM, Cadarache. Another key feature of ITER will be its ability to operate in the so-called High Confinement (H) mode, a particular condition of the system in which the central pressure is maximized, allowing an elevated fusion reaction rate. Although this regime of operation has been successfully achieved experimentally in most of the existent machines, the physical mechanism causing its triggering has not yet been given a full theoretical explanation. However, both theoretical models and experimental results are converging towards a theory where turbulence has a central role.
The need of a deeper understanding of plasma edge turbulence has led to a strong international effort in the development of 3D fluid simulation codes. Among the state-of-the-art numerical tools, TOKAM3X has been developed in the last years through the collaboration between IRFM and the M2P2 Laboratory (Mécanique, Modélisation et Procédés Propres) at Aix-Marseille University.
Until now, the modelling efforts of the international community have been focused on simple circular geometries, mainly because of computational constraints. TOKAM3X is now able to deal with a more realistic geometry. In particular, for the first time, simulations have been run using a diverted magnetic configuration, characteristic of almost all of the existing and future tokamaks, including ITER. This specific geometry, which presents a singularity in the magnetic field called X-point, has been shown in various experiments to be favourable for the triggering of the H-mode and for the control of the power exhaust. The aim of the recent TOKAM3X simulations is to gain an insight on the effect of the diverted magnetic geometry on the plasma edge turbulence.
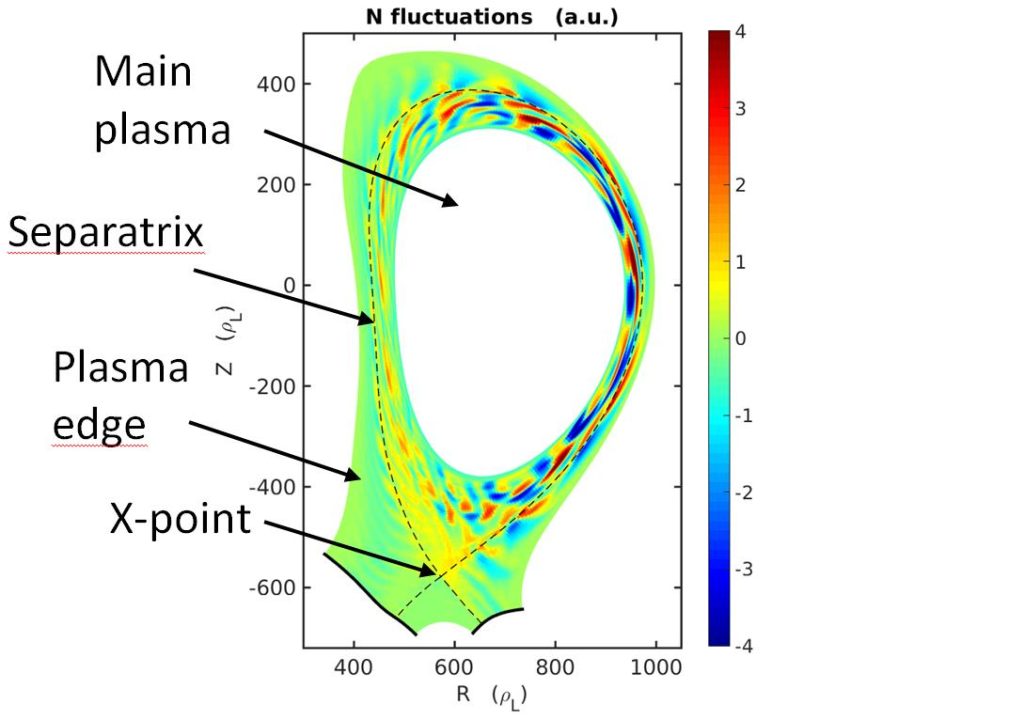
Simulation results show a strong coupling between large-scale flows and small-scale turbulence, highlighting the necessity to describe the problem with a first-principle multi-scale approach. The small-scale turbulence drives indeed macroscopic flows along the magnetic field lines, which in turn determine the heat-flux deposition pattern on the plasma wetted area. The main features of these macroscopic flows along magnetic field lines, such as their asymmetry due to the magnetic curvature, have been reproduced by the code, as well as the right order of magnitude of their amplitude.
The filamentary nature of turbulent structures, strongly aligned on the magnetic field’s direction, has been confirmed. Moreover, fluctuations are shown to be strongly elongated radially in regions where the field is almost toroidal, for example close to the X-point, as it has been recently observed experimentally by fast-imaging visible cameras. This information is an important guideline for the interpretation of measurements of turbulent fluxes in tokamak plasma edge.
A stronger confinement is found in divertor geometry with respect to the circular one, with a higher plasma pressure in the confined region. This suggests a favourable impact of the X-point geometry on the plasma stability, and could be an important step in the comprehension of the Low-to-High confinement transition mechanism.
Reference: Drive of parallel flows by turbulence and large-scale E × B transverse transport in divertor geometry
D. Galassi , P. Tamain, H. Bufferand, G. Ciraolo, Ph. Ghendrih, C. Baudoin, C. Colin, N. Fedorczak, N. Nace and E. Serre
Nuclear Fusion | 57:036029 | DOI: 10.1088/1741-4326/aa5332 (2017)
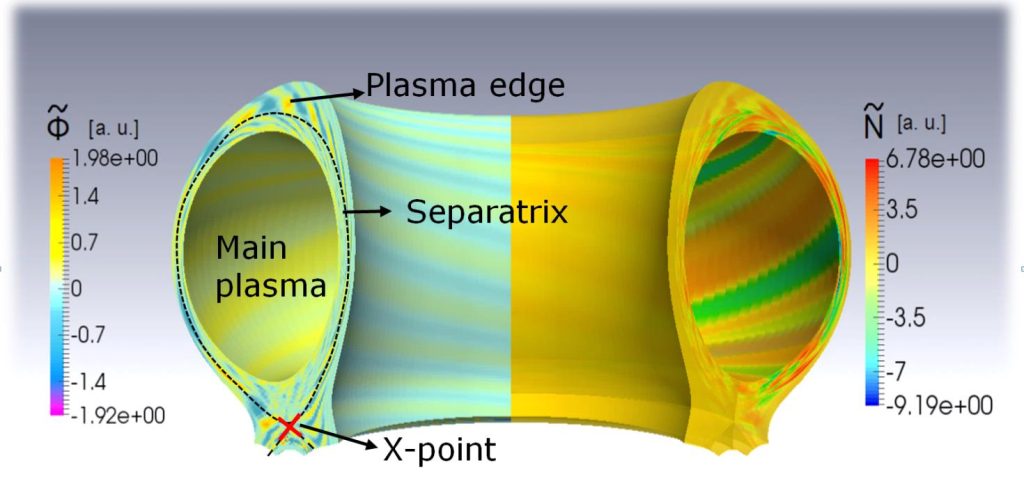